How to Dive Into Open Source Projects – A Beginner’s Guide
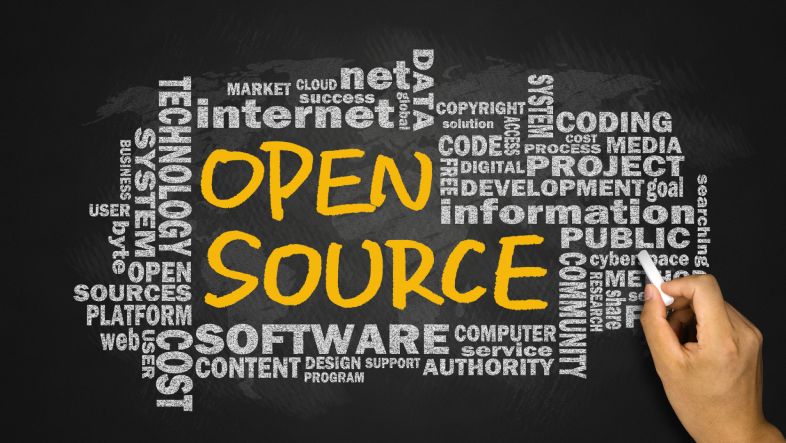
Introduction
Open source software, defined by its freely available source code, invites anyone to view, modify, and distribute it, fundamentally transforming software development through collaboration and transparency. This approach has catalyzed technological innovation, exemplified by achievements like the Linux operating system and the Firefox browser, and has bolstered security and quality through widespread developer participation. Beyond technical advancements, open source fosters an inclusive community, offers extensive learning opportunities, and provides economical solutions for individuals and organizations globally. As you explore how to contribute, remember you’re joining a movement that promotes shared knowledge and collective advancement in the tech world.
Embarking on your first open source project can be intimidating. You might worry about not knowing enough, making mistakes, or how to even get started. Fear not! Open source communities are built on collaboration and learning. This guide will walk you through the steps to get started and provide real-world examples of projects you can contribute to right away.
1. Choose the Right Project The first step is finding a project that interests you. This is crucial because your passion for the subject will sustain your interest and drive your contribution efforts. Look for a project that aligns with your skills and professional goals, or consider something that sparks your curiosity and passion.
Examples:
- Education: Moodle is a widely-used learning platform designed to provide educators with the tools to create personalised learning environments.
-
Sustainable Urban Planning: Streetmix is an interactive street section builder that allows users to design their ideal streetscapes in a simple, engaging way.
-
Healthcare: OpenMRS is an open-source platform that supports healthcare in developing countries. It’s a great project for developers interested in medical informatics.
-
Open Hardware: Arduino is an open-source electronics platform based on easy-to-use hardware and software. Arduino boards are able to read inputs and turn them into outputs.
-
Art and Design: Blender is a free and open source 3D creation suite that supports the entirety of the 3D pipeline—modeling, rigging, animation, simulation, rendering, compositing, and motion tracking.
2. Understand the Guidelines: Every project has a set of guidelines for contributors. Familiarize yourself with these to understand how to submit contributions, the coding standards expected, and the process for reporting bugs. This information is typically found in the project’s README or CONTRIBUTING.md files.
3. Start Small: Begin with small, manageable tasks. Look for issues labeled as “good first issue” or “beginner-friendly.” Starting small will help you get familiar with the project’s codebase and contribution process.
4. Communicate Effectively: Being an effective communicator is key in the open source community. Join the project’s community channels like Slack, Discord, or forums. Don’t be afraid to ask questions but ensure you’ve done your due diligence in trying to find the answer first.
5. Submit Quality Contributions: Whether fixing a bug, adding new features, or updating documentation, make sure your contributions are of high quality. Follow the coding standards, write clean and readable code, and include thorough documentation and test cases if applicable.
6. Learn and Improve: Open source is as much about learning as it is about contributing. Take feedback constructively and use it to improve your skills. Review other people’s contributions to learn different approaches and coding styles.
Conclusion
Contributing to open source can be a rewarding journey. Not only do you get to improve your technical skills, but you also contribute to projects that make a difference in the real world. So choose a project that resonates with you, and take your first steps into the vast world of open source!